It was thought until recently that electricity was created by the movement of electrons around a circuit. This worked fine for batteries but AC required a way to transfer energy across an isolation transformer where the primary electrons never touch the secondary winding electrons. We also know that electricity moves at nearly the speed of light, and as electrons are particles they would need a massive amount of energy to achieve this.
So we need to rethink how we can transmit electric light energy using magnons rather than electrons. As domestic electricity is alternating current [AC] it is really just a low frequency electromagnetic energy but subject to the same laws and restrictions as radio waves and sunlight rays.
To try and reconcile these requirements it is much easier to consider that magnons are at the inside heart of all types of electromagnetic vibrational energy which when introduced into matter molecules vibrates the inner nuclear magnetic moment and thus increase its temperature/pressure characteristics. To this end I wrote a blog on magnoflux http://electricmagnofluxuniverse.blogspot.com/
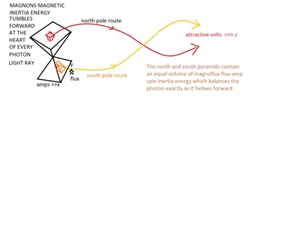